As the corresponding author, Paul A. Wender, co-founder of N1 Life and academician of Stanford University, published the results of cooperation with Professor Robert Waymouth and Professor Howard F. Chang in the well-known academic journal Journal of American Chemistry Society. The title is: "Organ- and Cell-Selective Delivery of mRNA In Vivo Using Guanidinylated Serinol Charge-Altering Releasable Transporters". This article introduces the new progress of Academician Wender's research group in developing organ- and cell-selective mRNA delivery vectors. The new generation of vectors, namely GSer-CARTs vectors, have the characteristics of extrahepatic targeting, controllability, and predictability, and achieve efficient in vivo delivery of circOVA and inhibit tumor growth by inducing immune response.
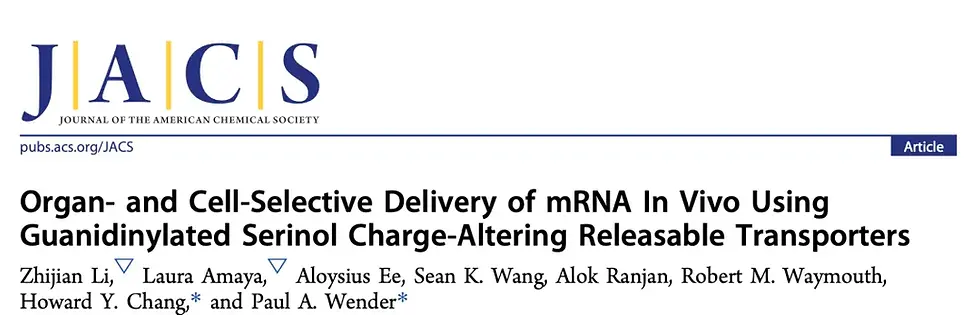
With the successful development of mRNA vaccine products, the field of RNA drugs has received widespread attention. However, to achieve the widespread application of RNA drugs, one of the major challenges is to develop a safe, effective and scalable delivery system that enables RNA molecules to pass through cell and organ barriers and release RNA at specific sites to exert their effects.
In the past few years, RNA delivery systems represented by lipid nanoparticles (LNPs) have made great progress. Lipid nanoparticles (LNPs) deliver RNA primarily to the liver when injected intravenously and can achieve local delivery when injected intramuscularly, but achieving efficient and selective delivery of RNA to other organs such as the lungs and lymphatic system remains a major technical challenge in the industry.
To achieve organ- and cell-selective delivery, one strategy is to modify the LNP surface with receptors that target specific cell surface receptors. This "active targeting" strategy can improve in vivo mRNA delivery to leukocytes, T lymphocytes, and brain cells compared to LNPs without targeting ligands. Targeting ligands that affect biodistribution can also be identified by in vivo library screening. In addition, the "corona effect", which is the modification of the LNP surface upon binding to ligands in the blood, can also effectively participate in organ-selective delivery. Another basic strategy to achieve efficient and selective delivery of mRNA is to design mRNA carriers that lose their cationic charge after in vivo administration. An example of this strategy, Charge-Altering Releasable Transporters (CARTs), was previously reported by the Chang, Levy, Waymouth, and Wender research groups. Wender's laboratory previously reported the early design, subsequent optimization and application of CART vectors. The vectors constructed by step-by-step simple assembly can effectively deliver a variety of RNA molecules including mRNA, siRNA, and siRNA.
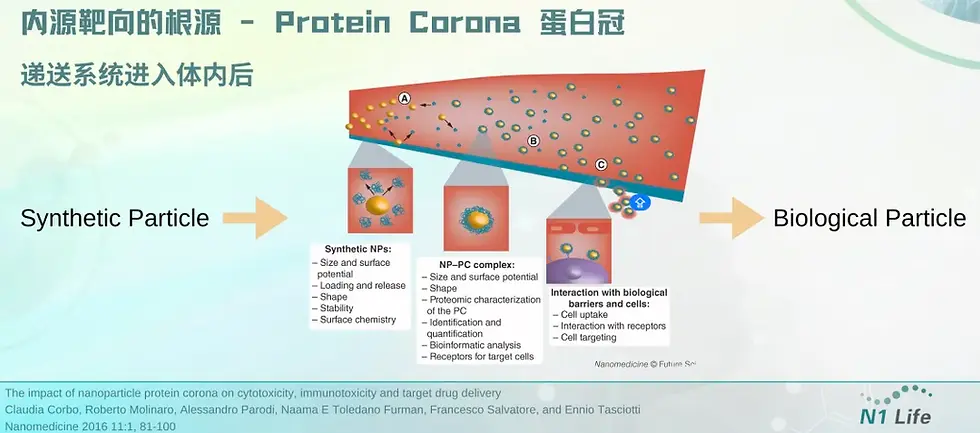
The key role of protein corona: mRNA delivery system undergoes "identity conversion" in vivo to achieve organ targeting | Dr. Janice Zang sharing at BioCon
Characteristics and principles
CARTs are block oligomers composed of an initiator (usually a hydroxyl nucleophile, used to initiate polymerization), one or more lipid modules, and a unique cationic α-ammonium ester module, in which the ammonium ester module can electrostatically complex with polyanionic RNA. Under low pH (<5.5), CARTs form a stable complex with mRNA; under physiological pH (~7.4), the cationic ammonium module undergoes oxygen-nitrogen acyl transfer and is irreversibly converted into a non-charged lactam byproduct, thereby triggering the release of polyanionic RNA. CARTs have been used to prepare CAR-NK cells, CAR-T cells, effective SARS-CoV-2 vaccines, and cure cancer and metastatic cancer in mouse models. Unlike LNP systems that are primarily delivered to the liver, CARTs exhibit extrahepatic tropism. For example, CARTs with the addition of fingolimod ligands exhibit selectivity for the spleen; while recently reported CARTs, in the absence of targeting ligands, exhibit significant spleen or lung selectivity. Therefore, CARTs technology provides a new strategy for achieving organ-selective delivery of mRNA. In this article, the Wender research team developed a new guanidine-containing transporter for RNA delivery, which is designed to degrade the guanidine group into neutral byproducts, thereby achieving efficient mRNA release and translation. Based on previous studies of CART carrier molecules, the authors replaced the commonly used charged ammonium cation group with guanidinyl serinol, so it is called GSer-CART.
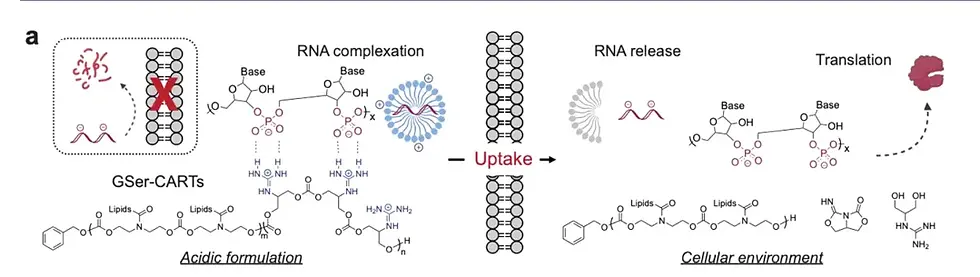
Figure [1] Biophysical properties and principles of GSer-CART and GSer-CART/mRNA complexes
Research process
First, the research team revealed a new mechanism through the study of model compounds: at low pH (<5.5), the guanidine group forms a double hydrogen bond ring with the carbonate group, and the transporter and mRNA form a stable packaged complex. At high pH, that is, at physiological neutral pH (~7.4), the guanidine group loses its positive charge, thereby eliminating its electrostatic binding with the phosphate group. The guanidine group in the complex can be quickly converted into an electrically neutral byproduct through a cyclization cascade reaction, allowing the two to separate and achieve the release of mRNA in cells.
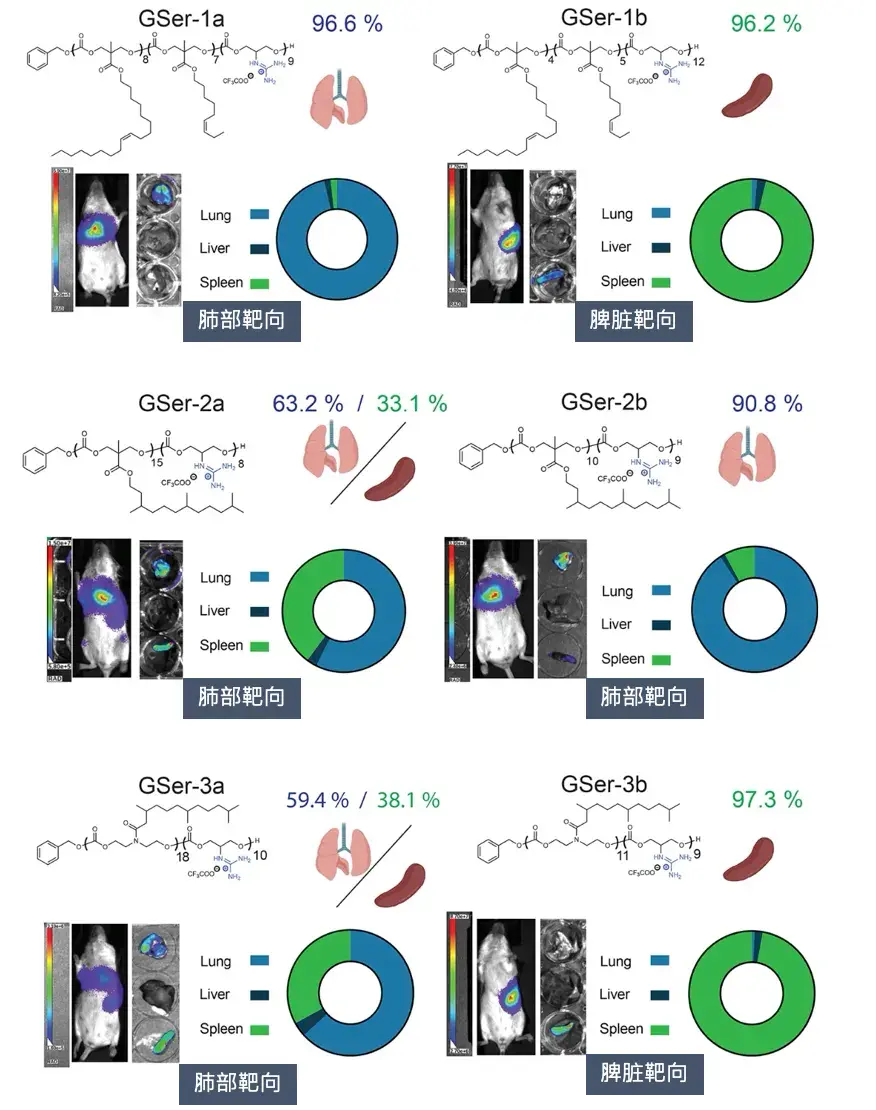
Figure [2] Adjustable organ targeting of GSer-CART in vivo
In subsequent in vivo experiments, the research team injected the six GSer-CARTs with the highest in vitro delivery efficiency into BALB/c mice intravenously. The results showed that CARTs with different side chain chemical structures and complexes with different formulation parameters would produce different organ preferences. The researchers found that a high charge ratio is beneficial for lung delivery, while a low charge ratio is beneficial for spleen delivery, which can achieve targeted delivery and protein expression in the lung (up to 96%) or spleen (up to 98%), respectively, indicating that adjusting the charge ratio can control the organ preference of GSer-CART. Compared with the LNP formulations that have been approved and used in multiple products and clinical studies, this feature of GSer-CART is much better than existing technologies. CART is rarely expressed in the liver, and its organ preference is not related to different routes of administration. It is a major breakthrough in the targeted delivery of mRNA to organs other than liver and muscle.
In this work, the application of GSer-CART vector technology has been extended to circRNA, i.e. circular RNA. Due to its unique closed circular structure, circRNA has no 5' and 3' ends that are susceptible to nuclease attack. This structural feature gives circRNA higher stability, making its half-life usually significantly longer than linear mRNA, so it has higher stability and more persistent protein expression, and exists in cells for a long time, exerting a continuous regulatory function. circRNA has great clinical potential. The stable presence of circRNA in body fluids (such as blood, saliva, and urine) makes it an ideal non-invasive liquid biopsy marker. Specific expression of circRNA is expected to become a powerful tool for early diagnosis of diseases, prognosis assessment, and treatment monitoring.
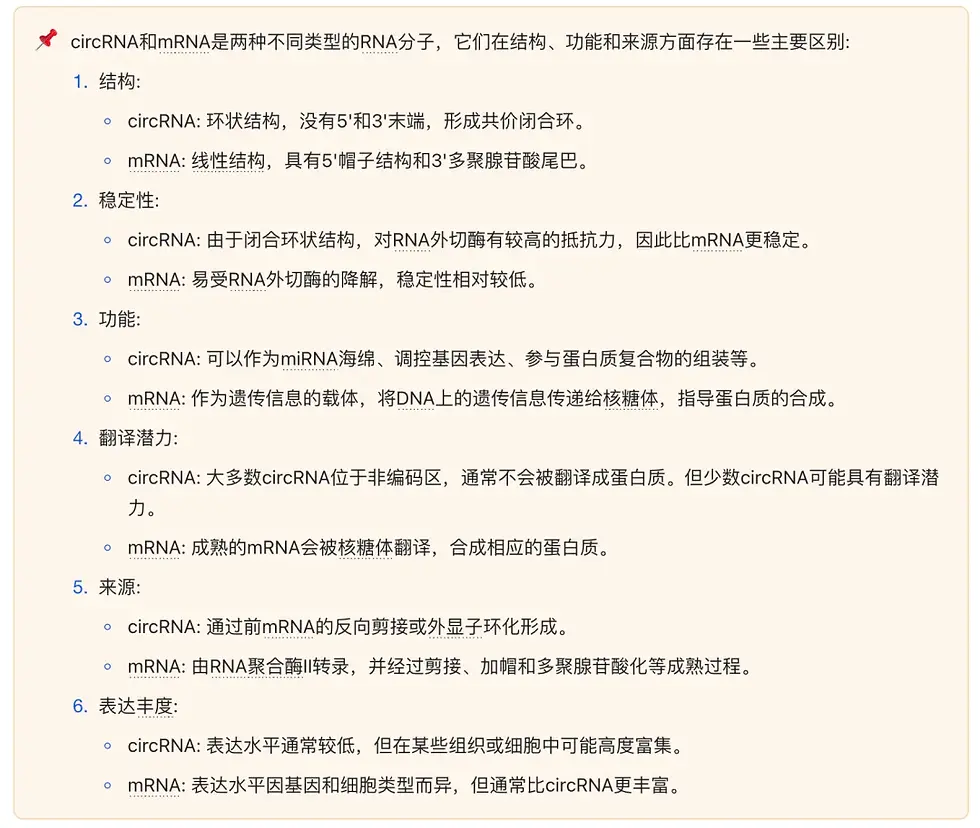
In addition, circRNA may also become a key target for new treatment strategies, such as circRNA vaccines and circRNA vectors. A past study published in PNAS showed that circRNA can also induce strong T cell immunity through the encapsulation and delivery of CART delivery vectors, and induced tumor clearance in a study of intraperitoneal injection after circRNA encapsulation with CART.
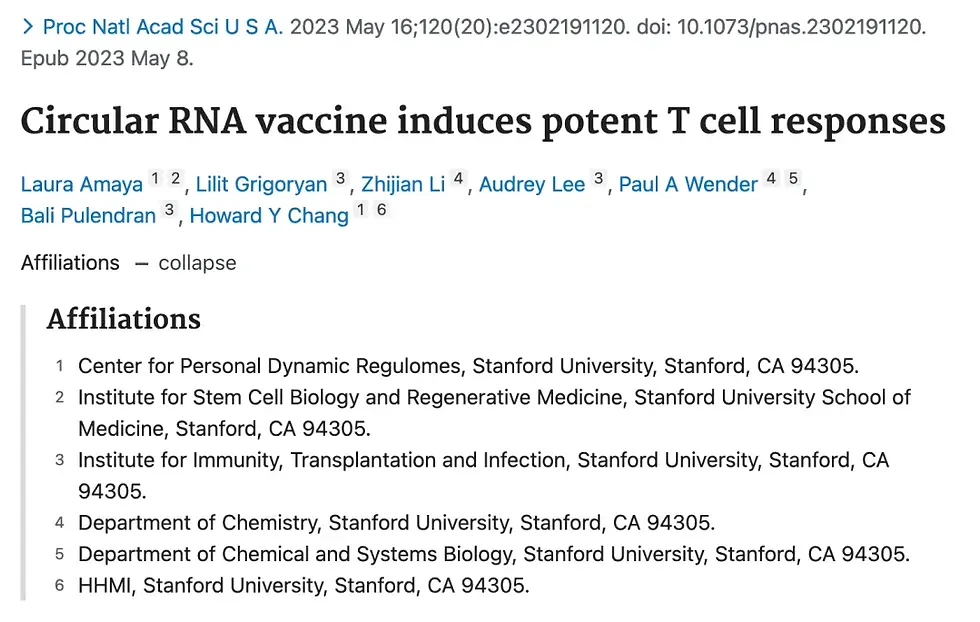
The researchers used two GSer-CARTs to deliver circRNA (circOVA) encoding ovalbumin (OVA). The results showed that GSer-3b/circOVA can induce strong T cell responses and significantly inhibit tumor growth in a mouse tumor model. The mouse model was established by subcutaneous injection of B16F10-OVA-Luc cells in C57BL/6J mice.
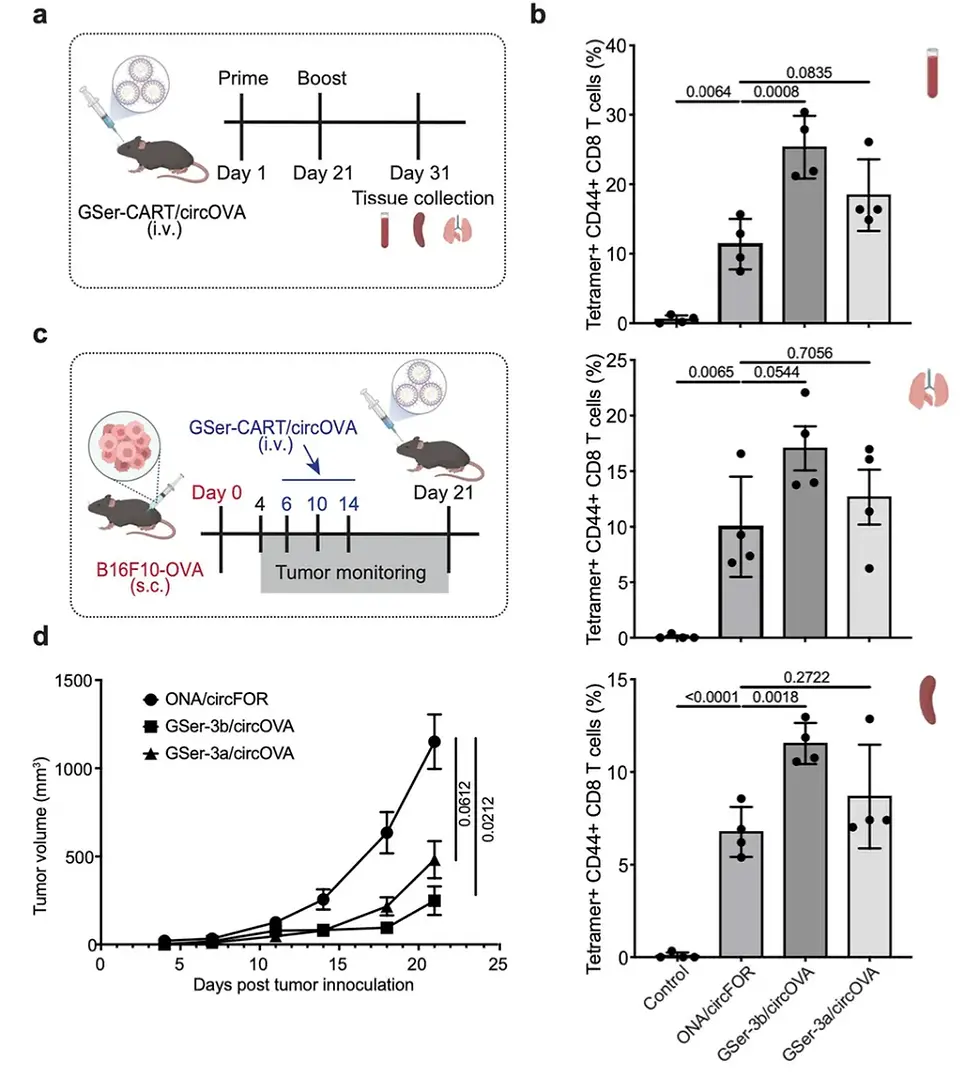
Figure [3] GSer-CART/circOVA complex can induce strong anti-tumor response and inhibit tumor growth
As shown in Figure [3]a, 5 μg of circOVA complexed with GSer-CART was injected retroretinaally on days 1 and 21. Antigen-specific CD8 T cell responses of blood, spleen, and lung T cells were analyzed by MHC class I tetramer staining 10 days after booster immunization. By bleeding mice 9 days after booster immunization to measure antibody responses, the researchers observed a significant increase in anti-Ova IgG in the GSer-3b/circOVA (spleen tropism) group. Notably, GSer-3b induced a strong T cell response in mice compared with the negative control. These findings emphasize that the use of GSer-CART complexed with circRNA (circOVA) encoding antigen sequences can effectively elicit antigen-specific CD8 T cell responses after immunization. Notably, the spleen-specific GSer-3b preparation produced the strongest antibody and T cell responses, which may be attributed to the spleen providing an ideal environment for activating dendritic cells and initiating antigen-specific T cell responses. The observed strong cytotoxic T cell responses prompted us to further explore the potential of GSer-CART/circOVA as a cancer vaccine. B16F10-OVA-Luc cells were subcutaneously inoculated into C57BL/6J mice, and GSer-CART/circOVA was injected intravenously at 6, 10, and 14 days after tumor injection. Both GSer-3a/circOVA and GSer-3b/circOVA complexes showed significant tumor growth inhibition compared with the control group (ONA-circFOR) (Figure 6d).
Figure [3]c shows a schematic diagram of tumor growth monitoring after inoculation of B16F10-OVA cells. On days 6, 10, and 14 after tumor cell inoculation, 5 μg of circOVA complexed with GSer-CART was injected retroretinaally, and tumor volume was monitored over 21 days.
Finally, we demonstrated that GSer-CART/mRNA complexes were non-toxic by blood metabolite and inflammatory cytokine analysis, and these complexes could be stored at different temperatures with different degrees of stability (Supplementary Note, Supplementary Figure 15). Together, these results demonstrate the delivery efficiency, selectivity, versatility, tolerability, and stability of GSer-CARTs for mRNA delivery.
Together, these results demonstrate the efficacy, selectivity, versatility, tolerability, and stability of GSer-CARTs for mRNA delivery.
Summary
This study demonstrates that novel CART vector technology has great potential for clinical application. By adjusting the chemical structure and formulation composition of GSer-CART, organ-selective (lung, spleen) and cell-selective (splenic macrophages) delivery can be controlled upon intravenous injection without the use of targeting ligands.
CART structural variations have previously been reported to exhibit organ tropism and are able to effectively deliver mRNA to multiple organs (lung, spleen, liver) with (active) or without (passive) the use of targeting ligands. However, the identification of novel formulation parameters for specific organs and cells remains largely empirical, and methods for predicting selective mRNA delivery remain limited. Previous literature suggests that the protein corona composition of mRNA nanoparticles plays a role in determining organ tropism, but the structural link between nanoparticle properties, protein corona formation, and tropism has not been well established. In this work, we observed that charge ratio controls organ tropism and that tropism correlates with the biophysical properties of the nanoparticles: lung-tropic nanoparticles exhibited higher surface charge at physiological pH compared to spleen-tropic nanoparticles, and these nanoparticles showed a more significant increase in size when incubated in mouse plasma due to their binding to proteins. The strategy of adjusting the charge ratio is partially similar to previous work on organ-tropic LNP systems, where the addition of cationic lipids promoted lung tropism, while anionic lipids promoted spleen tropism. However, neither size nor surface charge has been reported to correlate with organ tropism in LNP systems, suggesting that the organ targeting mechanisms of LNPs and CARTs may be different.
In addition, LNPs were prepared with various lipids and some LNPs were temporarily shielded by PEG lipids, thus masking the observation of size and surface charge differences between different organ-tropic LNPs. Further studies are needed to elucidate the underlying mechanisms between organ tropism and biophysical properties. Our current study highlights the critical importance of nanoparticle biophysical properties, especially the organ tropism controlled by charge ratio in a plasma environment. Importantly, these properties may serve as a cost- and time-effective strategy for screening future GSer-CART formulations to determine target organ tropism as a prelude to animal experimentation, and generally encourage the broader community to adopt this strategy to predict and/or design nanoparticles with specific organ and cell tropism.